Alpine Flora
The Flora of the Alpine Grassland – beautiful and endangered
by the Alpine Plant Conservation and Research Programme
The Alps – a European hotspot of phytodiversity
The European Alps harbour about 4450 vascular plant taxa, with a density of 2200 taxa per 10,000 km² (Aeschimann et al. 2011a). Together with some Mediterranean areas and the Pyrenees, we find the highest phytodiversity in Europe in this area. The most species rich areas of the Alps are in the west and the south, while the most endemic-rich areas are those that were glacier-free during the Pleistocene, as well as the south-west and the very eastern parts of the Alps (Tribsch & Schönswetter 2003, Tribsch 2004, Aeschimann et al. 2011b). While most of the central European grassland at low altitudes results from cattle grazing or mowing, we find in the alpine belt “native grassland” as an ancestral habitat of many of the widely cultivated grassland species found today.
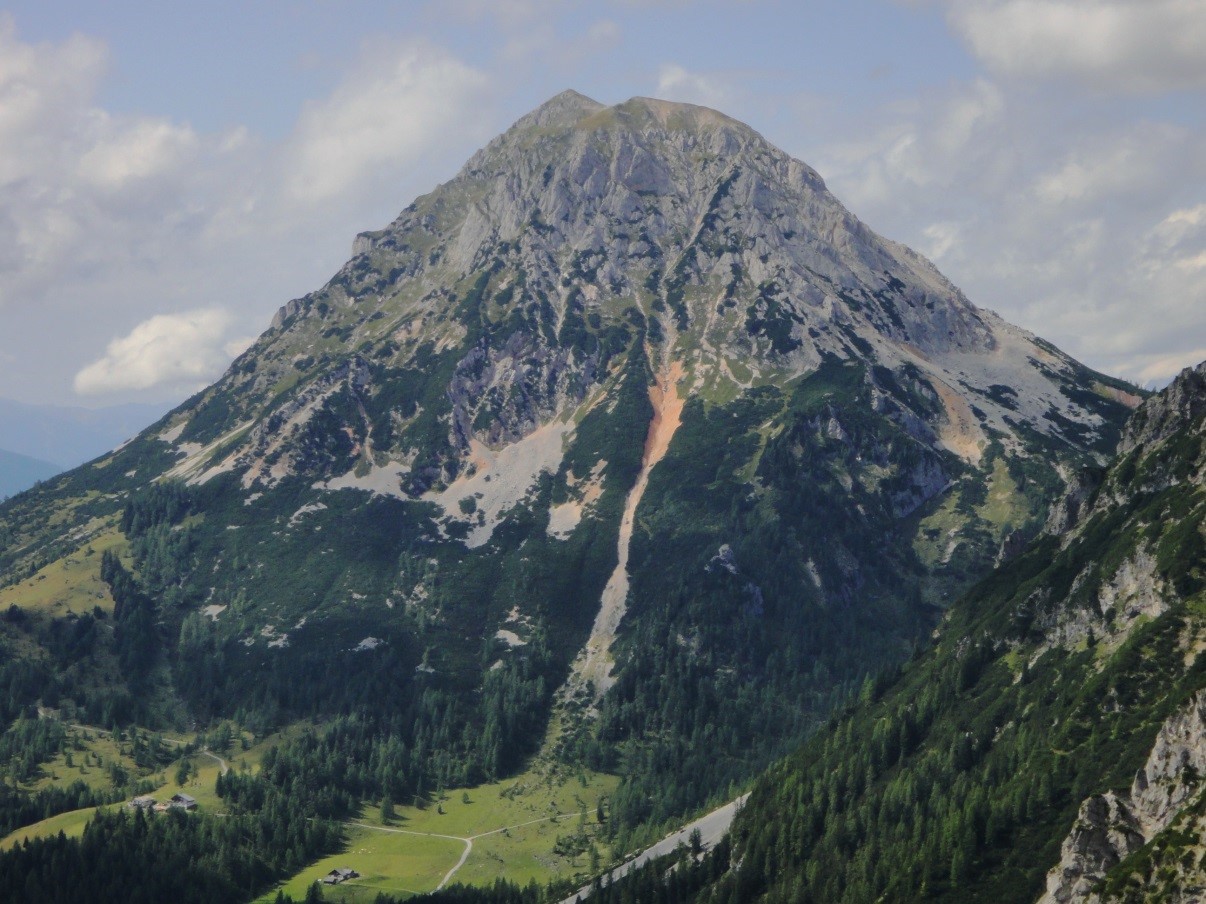
The ecological conditions for life are extreme here. With every 100 m increase in altitude the mean annual temperature decreases 0.7 degrees, leading to the characteristic vegetation zonation of high-montane forests, subalpine Krummholz with shrubs like Pinus mugo or Alnus alnobetula, and alpine grassland above the timberline. Elevation elongates the frost period, and reduces the vegetation period – the time during the year when plant growth is possible. In the same way, bedrock weathering, soil and humus development and especially microbial activities providing mineral nutrients for plant growth are reduced. Unlike arctic regions with similar conditions, solar radiation is high with an increasing amount of dangerous UV radiation and nocturnal back-up radiation with altitude. All life forms have to face huge differences between day and night temperatures and radiation. Precipitation is high, but the thin soils do not steadily provide water and nutrients to plant roots. Strong rain or snow melt cause floods that float the few nutrients away, while the soils dry out quickly in the hot radiation of sunny days.
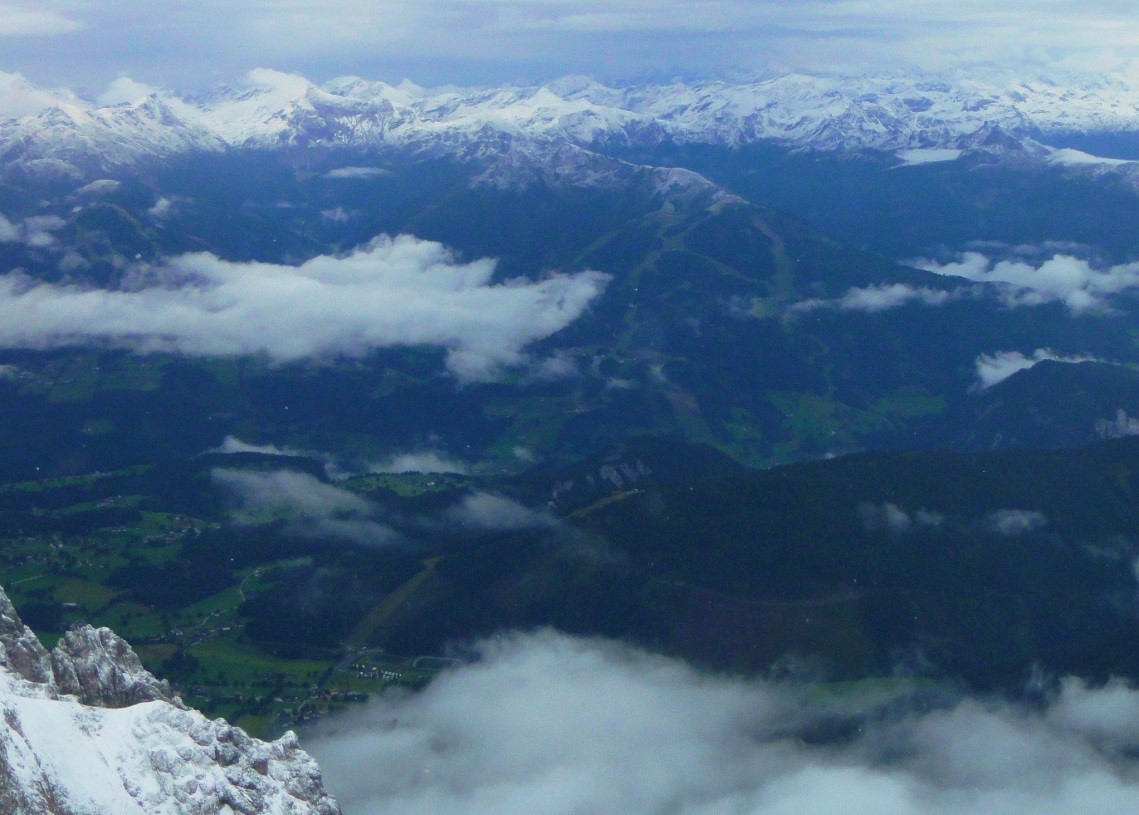
Seeds can be easily dispersed by wind in the Alps, but the winds are too strong for pollination and insect pollination predominates. Insects have evolved in conjunction with the most species-rich group in the Alps, with high rates of speciation and endemism, but with low individual numbers due to the harsh ecological conditions. Plants have to produce conspicuous flowers to attract insects in this harsh environment; one of the reasons we consider the alpine flora extraordinarily beautiful. Plants have also had to evolve a set of vegetative and regenerative adaptations to survive here on the physiological border of the Earth’s biosphere.
This short description of the natural conditions in alpine environments makes it clear that alpine life is a highly complex interactive system that has evolved over millions of years. Every link in the system has its own role in this ecological interplay, and the system is highly endangered if one link is lost – making Alpine belt ecosystems more sensitive to disturbance than most other ecosystems in the world.
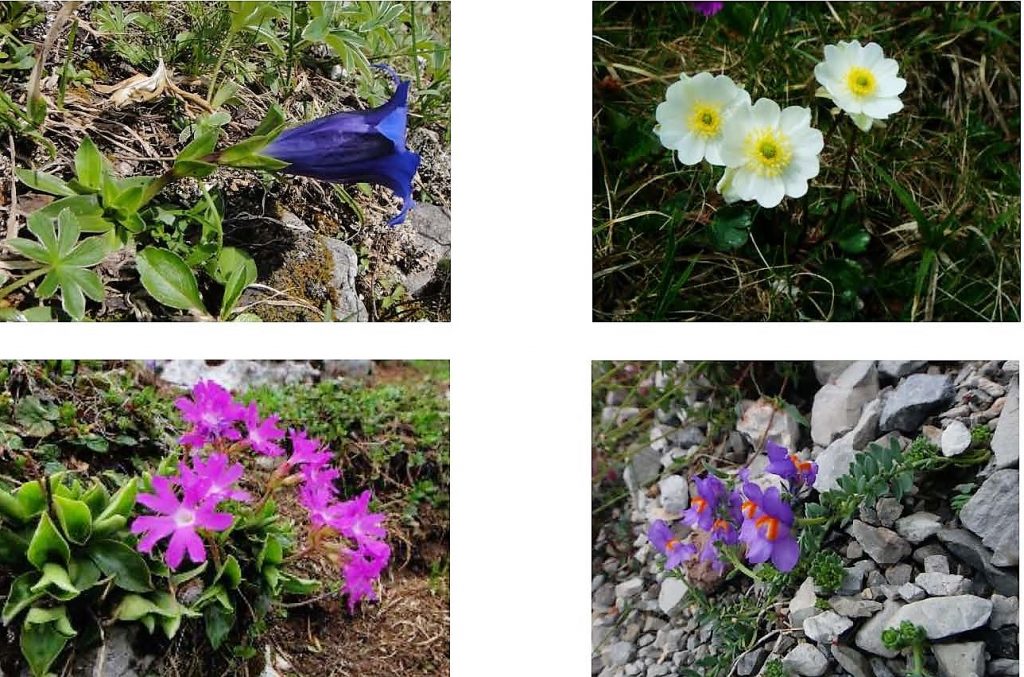
The endangerment of the flora of the Alps by habitat loss and tourism
The European Alps are a long-lasting region of pre-industrial land use. The pasture-like alpine grasslands invited ancestral people to bring cattle (mainly sheep and cows) to the alpine zone during the summer, and hey from the meadows was taken down to the valleys for winter feed. As result a diverse, mosaic landscape with montane meadows developed. While the alpine grassland expanded, the timberline and Krummholz-belt were relegated dozens of meters to lower altitudes. The impact on the native flora was low; many species of the alpine belt were adapted to light grazing by the natural pressure of wild chamois and Capricorns.
After the development of industrial agriculture in the second half of the 20th century the picture changed. Pre-industrial agriculture was not competitive any more, and tourism – notably winter sports – developed as a new source of income for the local population. This altered whole landscapes, robbed them of their grandiosity, and depreciated the Alps to the backdrop (Niedrist et al. 2009). The vegetation in winter sport areas is replaced, or at least strongly altered, with a clear decline in the sensitive alpine flora (Rusterholz et al. 2004, Wipf et al. 2005, Negro et al. 2010).
Winter tourism is, however, highly endangered by global warming. The answer of the tourism branch is to build up a giant and expensive infrastructure for artificial snow production (e.g. water basins above ski slopes), and move the ski slopes to higher altitudes or to northern exposures. The impact of the tourism industry to the nature of the Alps has reached a new level at the 21th century.
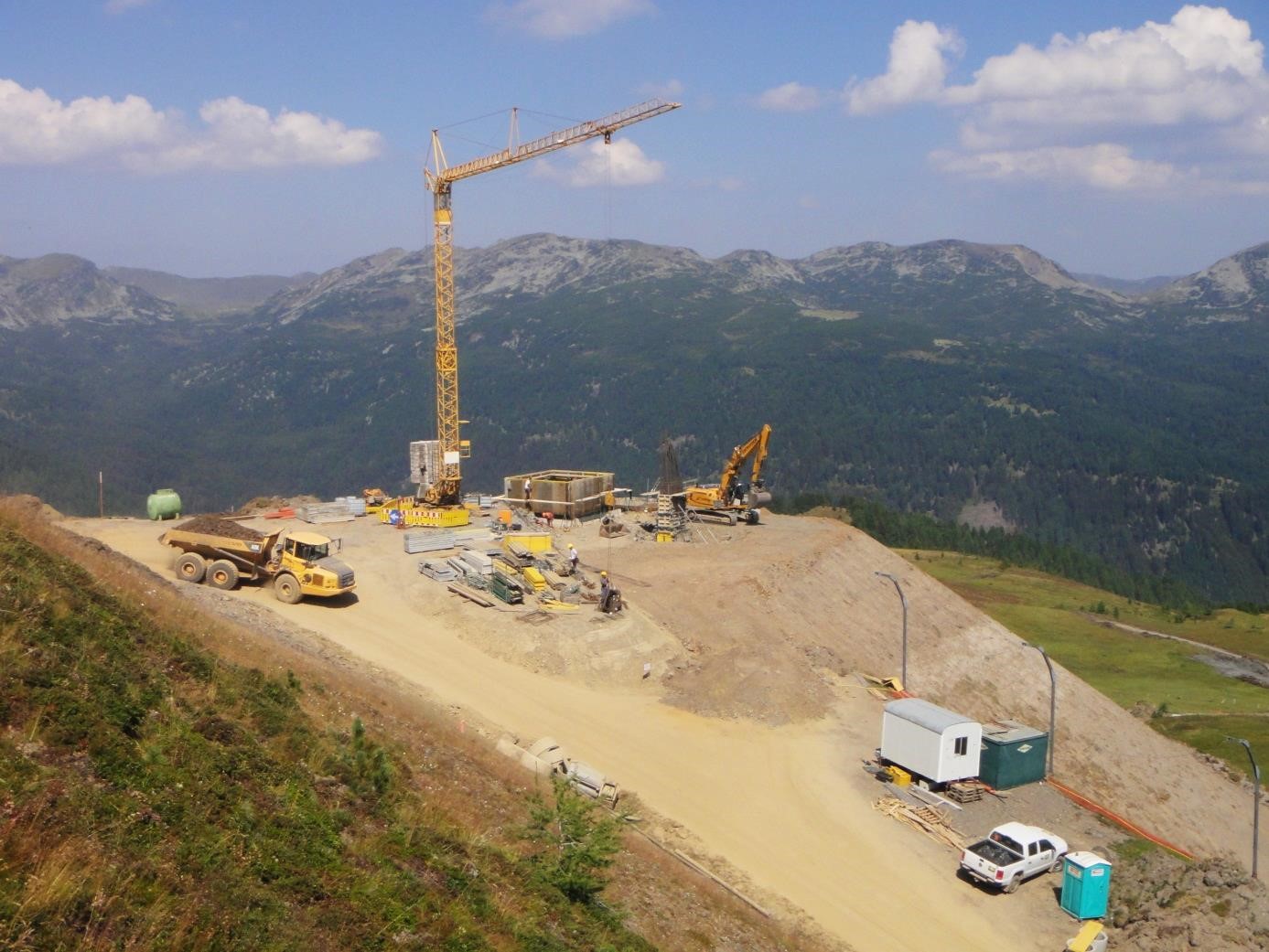
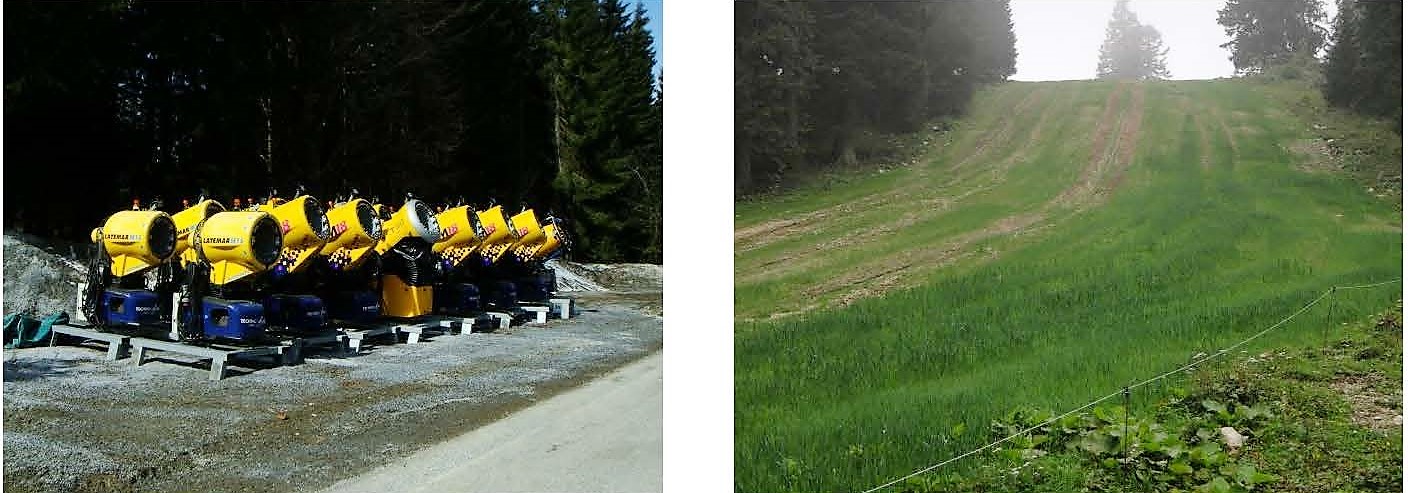
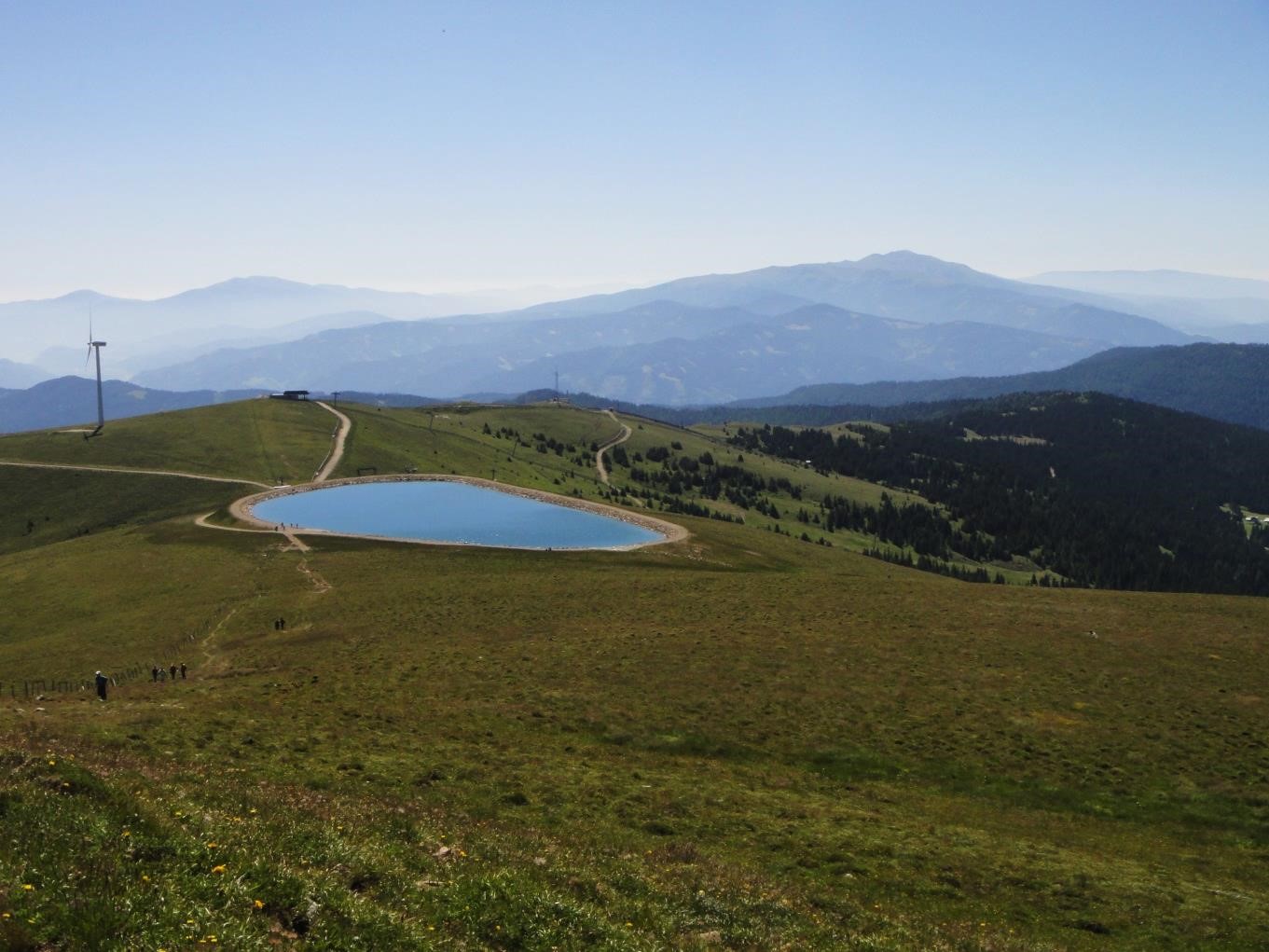
As a result, farmers concentrate their cattle in the remaining areas, or give up completely, losing their good, accessible pastures and find new jobs in tourism. Both have negative effects on the alpine flora. High densities of cattle facilitate the negative effects of grazing on the vegetation, preventing many species from producing seeds, with long-term negative effects on population development. Threatened species are more sensitive to the impacts of over-grazing e.g. dwarf shrubs, cushion and wetland plants. In areas were livestock are completely removed, high montane forest and Krummholz recolonise from lower altitudes, reducing the habitat of alpine grassland species.
Climate change – the main threat of the alpine flora
The example of winter sports areas show the indirect impact of global warming on alpine plants through exacerbated habitat loss. The direct effects of climate change on alpine ecosystems are even greater, and will be the much bigger challenge for nature conservation in the 21st century. Since the 1990s, the mean temperature of the Alps has been well above the long-term mean, and far beyond the global mean (Beniston 2006). Climate scenarios predict a temperature increase of 4 to 4.5 degrees by 2100, with less precipitation in the Western Alps and slightly more precipitation in the Eastern Alps (Heimann & Sept 2000, Beniston 2005). In addition, there will be less snow cover and a reduction of permafrost across the Alps (Beniston 2006, Harris et al. 2009).
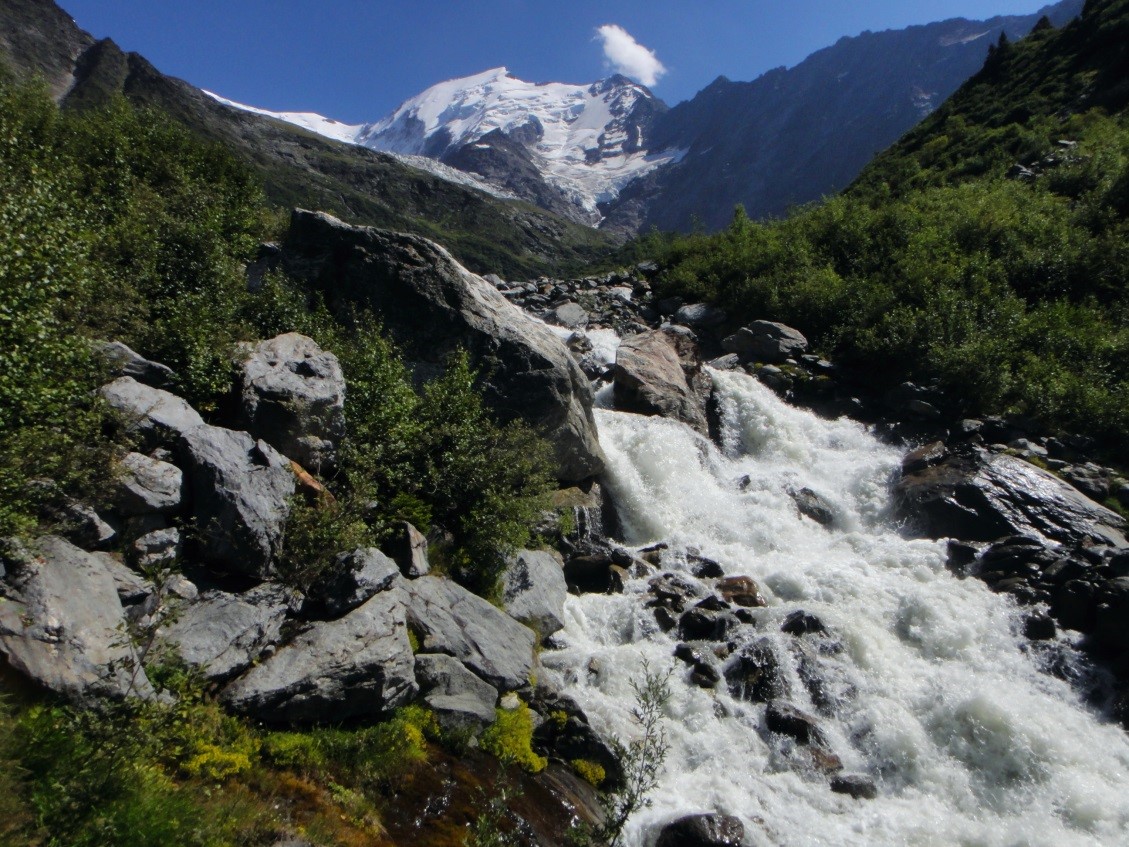
Climate change will affect global biodiversity (Malcolm et al. 2006). According to the vegetation sensitivity index (Seddon et al. 2016), arctic and alpine ecosystems are most sensitive to climate change. For a long time, the shrinking of alpine glaciers was used as the most visible and spectacular indicator of climate change (Haeberli et al. 2007). The reaction of plant cover is different (Dirnböck et al. 2003, Erschbamer 2007) and not easy to explain (Theurillat & Guisan 2001, Rixen et al. 2014). According to most predicted scenarios, a general up-shift of plant populations (Pauli et al. 1996, Erschbamer et al. 2009), vegetation units (Brzeziecki 1995) and the timberline (Gehrig-Fasel et al. 2007) can be expected, as well as exceptions for individuals and populations that use microclimatic heterogeneity of a particular habitat to remain at the same altitude (Maclean et al. 2015). It is also known, that small range species with small populations are more affected by climate change (Ohlemüller et al 2008). Even if we consider that the retreat of the glaciers provide additional habitats for alpine and subnival species in a “cooled” glacier mesoclimate, all summits are finite and the cone shape of summits results in a permanent reduction of the available surface area at higher altitudes.
In our project, we want to get a deeper insight into plant reactions to these pressures in light of increasing future endangerment, from reproduction parameters like seed production and germination strategies to the ecological level.
References
Aeschimann, D., N. Rasolofo & J.-P. Theurillat (2011a). Analysis of the flora of the Alps. 1: historical account and biodiversity. Candollea 66: 27-55.
Aeschimann, D., N. Rasolofo & J.-P. Theurillat (2011b). Analysis of the flora of the Alps. 2: biodiversity and chorology. Candollea 66: 225-253.
Beniston, M. (2005). Mountain Climates and Climatic Change: An Overview of Processes Focusing on the European Alps. Pure and Applied Geophysics 162, 1587–1606.
Beniston, M. (2006): Mountain Weather and Climate: A General Overview and a Focus on Climatic Change in the Alps. Hydrobiologia 562: 3–16.
Brzeziecki, B., Kienast, F. & Wildi, O. (1995). Modelling potential impacts of climate change on the spatial distribution of zonal forest communities in Switzerland. Journal of Vegetation Science 6: 257–268.
Dirnböck, T., Dullinger, S., Grabherr, G., 2003. A regional impact assessment of climate and land-use change on alpine vegetation. Journal of Biogeography 30, 401–417.
Erschbamer, B. (2007). Winners and Losers of Climate Change in a Central Alpine Glacier Foreland. Arctic, Antarctic, and Alpine Research 39, 237–244.
Erschbamer, B., Kiebacher, T., Mallaun, M. & Unterluggauer, P. (2009). Short-term signals of climate change along an altitudinal gradient in the South Alps. Plant Ecology 202, 79–89.
Gehrig-Fasel, J., Guisan, A. & Zimmermann, N. E. (2007). Tree line shifts in the Swiss Alps: Climate change or land abandonment? Journal of Vegetation Science 18, 571–582.
Haeberli, W., Hoelzle, M., Paul, F. & Zemp, M. (2007). Integrated monitoring of mountain glaciers as key indicators of global climate change: the European Alps. Annals of Glaciology 46, 150–160.
Heimann, D. & Sept, V. (2000). Climate Change Estimates of Summer Temperature and Precipitation in the Alpine Region. Theoretical and Applied Climatology 66, 1–12.
Harris, C., et al. (2009). Permafrost and climate in Europe: Monitoring and modelling thermal, geomorphological and geotechnical responses. Earth-Science Reviews 92, 117–171
Maclean, I. M. D., Hopkins, J. J., Bennie, J. J. & Lawson, C. R. (2015). Microclimates buffer plant community responses to climate change. Global Ecology & Biogeography, 24, 1340–1350.
Malcolm, J.R., Liu, C., Neilson, R.P., Hansen, L., Hannah, L., 2006. Global warming and extinctions of endemic species from biodiversity hotspots. Conservation Biology 20, 538–548.
Negro, M., Isaia, M., Palestrini, C., Schoenhofer, A. & Rolando, A. (2010). The impact of high-altitude ski pistes on ground-dwelling arthropods in the Alps. Biodiversity and Conservation 19, 1853–1870.
Niedrist, G., Tasser, E., Lüth, C., Dalla Via, J. & Tappeiner, U. (2009). Plant diversity declines with recent land use changes in European Alps. Plant Ecology 202, 195–210.
Ohlemüller, R., Anderson, B.J., Araujo, M.B., Butchart, S.H.M., Kudrna, O., Ridgely, R.S., Thomas, C.D., 2008. The coincidence of climatic and species rarity: high risk to small-range species from climate change. Biology Letters 4: 568-572.
Pauli, H., Gottfried, M. & Grabherr, G. (1996). Effects of climate change on mountain ecosystems – upward shifting of alpine plants. World Resource Review 8, 382–390.
Rixen, C., Wipf, S., Frei, E. & Stöckli, V. (2014). Faster, higher, more? Past, present and future dynamics of alpine and arctic flora under climate change. Alpine Botany 124, 77–79.
Rusterholz, H.-P., Müller, S. W. & Baur, B. (2004). Effects of rock climbing on plant communities on exposed limestone cliffs in the Swiss Jura Mountains. Applied Vegetation Science 7, 35–40.
Seddon, A. W. R., Macias-Fauria, M., Long, P. R., Benz, D. & Willis, K. J. (2016). Sensitivity of global terrestrial ecosystems to climate variability. Nature 531, 229–232.
Theurillat, J. & Guisan, A. (2001). Potential Impact of Climate Change on Vegetation in the European Alps: A Review. Climatic Change 50, 77–109.
Tribsch, A. 2004. Areas of endemism of vascular plants in the Eastern Alps in relation to Pleistocene glaciation. Journal of Biogeography 31, 747–760.
Tribsch, A. & Schönswetter, P. 2003. Patterns of endemism and comparative phylogeography confirm palaeo-environmental evidence for Pleistocene refugia in the Eastern Alps. Taxon 52, 477–497.
Wipf, S., Rixen, C., Fischer, M., Schmid, B. & Stoeckli, V. (2005). Effects of ski piste preparation on alpine vegetation. Journal of Applied Ecology 42, 306–316.
Corresponding author: christian.berg@uni-graz.at